Current Student Projects:
We are a spectroscopy group; with other words, we hit stuff with lasers and get excited about what happens! And even better, sometimes we understand why it happens. We have several exciting projects for undergraduate and graduate students. If you would like to learn more, please do not hesitate to email me.
Our research is inter- and cross disciplinary; the emerging materials studied in our lab are mainly synthesized by our great collaborators around the world. We closely work with chemical engineers (Yale, ColState), biomedical engineers (UNT), electrical engineers (UNT), material engineers (UNT), material scientists (UCL), chemists (Boston College, University of Warsaw, UNT, Rutgers), and physicists (UNT, U Arkansas). These outstanding scientists provide us with the most exciting emerging materials, complemented by the less exciting stuff we buy or cook in our group 😊. Our current projects are fluent, and the list below will be out-of-date frequently, so please feel free to email me for further information and to learn about the coolest current projects that we are working on.
Our research invovles advanced spectroscopy, data processing, cleanroom fabrication, and even chemical synthesis. This is a large field and undergraduate students will need time to master some of these skils. So if you are an undergraduate please think about joining a research group early on (Sophmore/Junior). We do not accept Seniors in their final 9 months at UNT; this is not enough time to conduct research in our group!
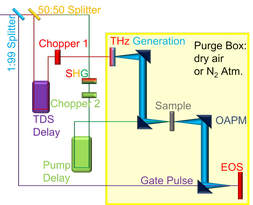
Simplified depiction of our main Spectrometer, about 20 mirrors were omitted for clarity. (More details in our recent APL Photonics publication)
Disclaimer: We are experimental spectroscopists; we built our own spectrometers! When you feel confused by the biological, chemical, and engineering details in the projects below, keep in mind: the details of the materials are exciting but the main expertise that you will acquire in our group is experimental light matter interaction. You will align 30 mirrors to guide three laser beams, over a distance of 2 meter each, to overlap at a 100 µm spot with a length error tolerance of less than 10 ps, or 0.015 % of the total length! You will learn what resonances, reflections, standing wave interference in samples, lock-in amplification, basic read-out electronics, noise, and laser-spectroscopy in general is. We work hard to get ever better spectrometers and collect spectra with unmatched signal to noise ratio, which is needed to explore the most challenging materials. As a fair warning: this includes long hours in dark rooms, just to go home frustrated because the “improvement” resulted in a factor 2 lower signal to noise ratio. But when it works, it’s pretty cool. This is also the reason why I expect any student who wants to perform undergraduate research (thesis/capstone) with me to reach out during their junior year (or earlier) to give us both time to figure out if this is a good fit!
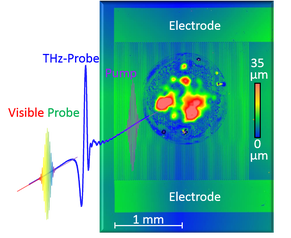
Our groups main interest lies in understanding conductivity and photoconductivity in materials for renewable energy applications. Silicon solar cells were demonstrated in the late 50s and since the 80s they are pretty well understood; and of course, utilized for solar-energy production. But they reached their limits and are exhaustively optimized; the past was the century of Silicon; our research will explore what the material of the current century will be. We research on the forefront of material spectroscopy: studying 2D materials, perovskites, thin-film semiconductor, and basically anything exciting we can get our hands on 😊. THz spectroscopy, and especially OPTP is used to understand how materials (photo) conduct charges. Understanding the underlying physics, the interactions of electrons with phonons, and the formation of quasi particles is crucial to design even better materials.
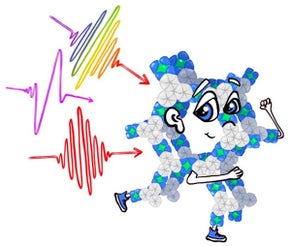
Illustration of Multi-wavelength ultrafast pump probe spectroscopy on metal organic frameworks. (published in Chemical Reviews, 2022, 122, 1)
Conductivity in Metal Organic Frameworks (MOFs): MOFs are chemical compounds formed by metals and organic linkers. The resulting framework is microporous, meaning mainly empty. The resulting solid material has a density of a few tens of kg/m3; two orders of magnitude lighter than Silicon. This provides unique potential for applications in wearable electronics and for space applications. But to leverage these advantages we first need to understand how MOFs conduct electricity? Two orders of magnitude fewer atoms per volume than Silicon, also means two orders of magnitude fewer electrons; and even more crucial fewer wave-function orbital overlap! So how does an electron get around such a sparse material? What are the electron highways connecting the metal nodes and allowing for long range charge transport? What limits conductivity and how can we overcome these limitations? And how can we reduce the defect density, or at least the impact of defects on the conductivity? Join us to find out!
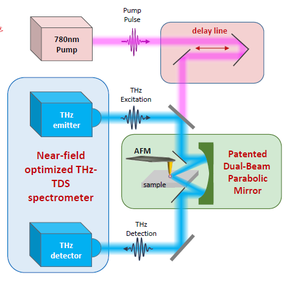
Scattering near field optical microscopy (SNOM) leveraged the local enhancement of light close to an atomic force microscope tip. SNOM, squeezes light into a small location, defined by the tip radius, not by the wavelength. Applying this technique into the THz range allows us to measure THz properties with 100 nm resolution, which is 1000 times smaller than the wavelength used to measure it. We recently acquired a commercial THz-SNOM, combined with pump-probe and spatial DC-conductivity measurements. With this cool new toy at hand, we now want to understand new and exciting physics. We have graduate and undergraduate research projects that focus on modeling, simulation, and experimental verification of light-matter interactions on the nanoscale. We will explore core-shell materials, interfaces, and buried layers. Understanding the physics that defines devices!
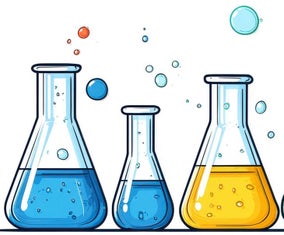
Development of novel materials for light harvesting is driven by a detailed understanding of ultrafast photophysics. We are experts in the latter and more recently started in the chemistry part of this challenge. Our group is appointed by courtesy with the Chemistry department and we are actively seeking students who are eager to drive these cross disciplinary projects. We have access to wet-chemistry and are working on MOF and Nanoparticle synthesis. We are eager to understand the interaction between these two materials. MOF coated nanoparticles for example are particularly interesting for future solar chemistry. These core-shell materials might harvest the best of both worlds and allow for tuning both parameters independently.
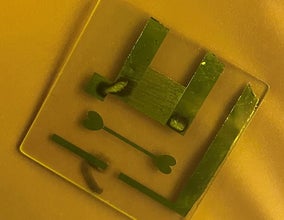
We are developing THz spectrochemistry, a technique that measures THz properties under adjustable Fermi levels. A three-electrode cell is used to change the Fermi level, while we measure conductivity and photoconductivity. Both will depend on the Fermi level relative to Band and Orbital energies. Understanding this dependency provides insight into what energy levels are involved in the photophysics; what trap states can limit carrier lifetime, and what molecular orbitals facilitate charge transfer.
The student projects will include: cleanroom fabrication, COMSOL simulation, and Spectroscopy. A student will be invovled in all or some of these fields and learning skills that are highly relevant for future research and industrial application.
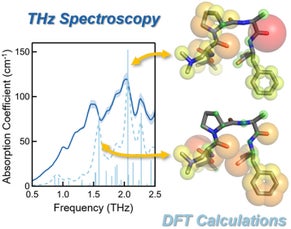
Distribution of Vibrational energy in a peptide, published in JPCL (We haven't done it yet for MOFs, so this figure could show your data!)
Vibrational Spectroscopy of Metal Organic Frameworks (MOFs): THz photons correspond to energies of 0.4-40 meV. At these very low energies excitations are related to delocalized vibrational/transversal modes. Basically, every atom in the MOF is excited when THz photons come around. This collective, delocalized motion provides unique insight into the dynamic structure of the material. It can tell us about the chemical interactions, the lattice symmetries, and the MOF-guest interactions. Understanding what happens at these frequencies is challenging; but rewarding. The weak interactions probed with THz are caused by the same forces that govern the performance of the material itself. The ps time scale of THz spectroscopy is the same time scale on which MOFs “work”, including gas adsorption, catalysis, and structural changes. Understanding THz spectra means understanding the material physics! We combine spectroscopy with density functional theory orders of magnitude more accurate than the “gold standard” in the field. If you want to understand chemistry from a physicist’s perspective, join us.
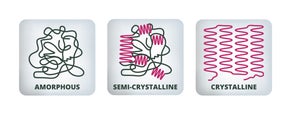
Adobe Stock Image: The goal of our research is to use THz to decipher which of these polymorphs a given polymer is in. Again, a new project that in future will feature the newest results from our students.
THz spectroscopy of Polymers: Polymers are all around us. They are omnipresent in commercial applications. And they are surprisingly little understood? They undergo phase transitions (glass transition) and aging that alters their properties, significantly limiting their reliability in applications, or providing new exciting applications. Together with our partners we strive to understand the physics that determine polymer properties. Polymers are formed by weakly interacting organic compounds (like MOFs). The forces holding a polymer together can be probed with THz spectroscopy. We will measure polymers under defined conditions to understand how the opto-mechanical properties change with temperature, water content, and aging. Understanding what happens in polymers will help us (and our collaborators) to improve them for specific applications. If you can get as excited as we are about rubber, join us!
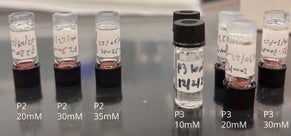
Peptide caused gel formation, published in Jacquelin (Jax)'s senior thesis at Yale-NUS. This project is near completion, but some follow up projects might be possible.
Understanding Hydrogel Formation with THz Spectroscopy: Hydrogels are formed by a variety of biological materials especially by proteins and peptides. The formation of these gels is crucial for cell function and imaging of living cells, a process called phase separation in biology. The underlying mechanism of this process is not yet understood and we work with colleagues at Yale, the National University of Singapore (NSU), and Avril University to understand what the forces are that form these gels. Understanding the underlying physics will be crucial for future biomedical applications, ranging from targeted drug delivery to understanding cell function. From a physicist perspective the core questions are, how does a few millimolar of a peptide force the whole liquid (50 mol) to form a gel? What interactions allow each peptide to “hold” thousands of water molecules in the gel? Why do some peptides display such a caging ability and other don’t? And how does this change the THz response? Read more in our upcoming publication when we hopefully figured this out.
I am sure I forgot a few projects, but I am also pretty sure that if you read all the previous points, you are in information overload anyway. So if you are interested to learn more about our research, please do not hesitate to email me.
Create Your Own Website With Webador